What a Fusion Energy Breakthrough Means for Green Power
Four times now, researchers have produced a fleeting burst of fusion energy, an encouraging sign for making this zero-carbon energy source a reality.
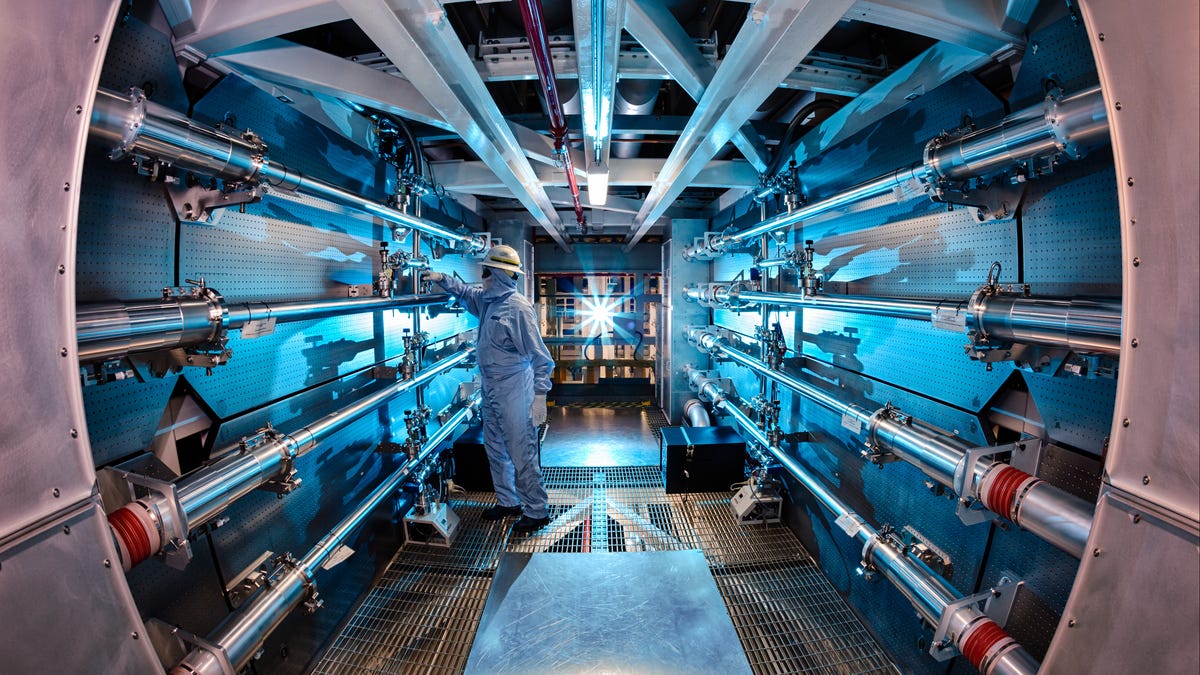
A technician adjusts an optic inside the preamplifier support structure at Lawrence Livermore's National Ignition Facility.
Scientists at Lawrence Livermore National Laboratory who achieved a major fusion milestone a year ago have repeated it three times more. Each experiment used 192 lasers to ignite a fusion reaction that for a fleeting moment produced more energy than was used to trigger it.
Fusion powers the sun, and humans reproduced the process more than 70 years ago to power thermonuclear weapons. The scientific and engineering challenges of a controlled fusion reaction, though, are formidable. The repeated successes by the scientists at LLNL's National Ignition Facility, gradually increasing the laser power that causes a tiny fusion fuel pellet to implode, are important steps of progress toward sustained, controlled fusion.
"Higher laser energy can help achieve a more stable implosion, resulting in higher yields," said Jean-Michel Di Nicola, a NIF leader, in a statement after the fourth fusion success on Oct. 30.
But what do the experiments mean for science and for the dream of a new energy source that would power our homes and cars without releasing any carbon dioxide?
In short, it's fine to applaud the NIF achievements, but they don't mean a green energy revolution is imminent. It'll still be years before fusion power progress bears fruit — likely a decade or so — and it's still not clear if fusion will ever be cheap enough to radically transform our power grid. Continuing today's investments in solar and wind is critical to combating climate change.
Commercial fusion ventures applauded the NIF experiment and have made gradual progress since then. Commonwealth Fusion Systems opened a new headquarters in Devens, Massachusetts, where it's building an experimental reactor designed to produce power. Tokamak Energy and General Fusion announced new facilities to be built near Oxford in the UK. Microsoft has agreed to buy fusion power from a Helion Energy plant called Constellation scheduled to go online in 2028. Other startups like Zap Energy and TAE Technologies are touting progress, too.
Here's a look at what's happened and what's still to come.
What is fusion?
The National Ignition Facility uses infrared and ultraviolet laser light to produce X-rays in a chamber with a peppercorn-sized fusion fuel pellet.
Fusion occurs when two lighter elements like hydrogen or helium merge into a single, heavier element. This nuclear reaction releases a lot of energy, as exhibited by the biggest fusion furnace around, the sun.
It's harder to get fusion to occur on Earth, though, because atomic nuclei are positively charged and therefore repel each other. The sun's enormous mass produces tremendous pressure that overcomes that repulsion, but on Earth, other forces are required.
Two general approaches to squeeze atoms together and produce fusion are called inertial and magnetic confinement. Inertial confinement usually uses lasers to zap a pellet with a lot of power, triggering an explosion that compresses the fusion fuel. That's the method NIF uses.
The other approach uses magnetic fields. It's more widespread among companies trying to commercialize fusion energy.
What did the experiment at NIF accomplish?
In December 2022, a NIF experiment crossed a critical threshold for fusion where the energy that the fusion reaction generated — 3.15 million joules — exceeded the 2.05 megajoules from the lasers to trigger the reaction. Because much more energy is required to run the lasers, though, the reaction overall is highly inefficient.
Fusion researchers denote the ratio of output energy to input energy with the letter Q, and the December 2022 reaction was the first time a fusion reaction surpassed Q = 1. On July 20, Oct. 8 and Oct. 30 of this year, NIF repeated its success in which Q was greater than 1. The Oct. 30 experiment used a record amount of laser power, 2.2 megajoules, an improvement that's difficult since the lasers can destroy the optical equipment that guide their light.
"It's all about the control of the damage," said NIF operations leader Bruno Van Wonterghem in a statement. "Too much energy without proper protection, and your optics blow to pieces."
Fusion reactors will have to reach a threshold of Q = 10 before energy generation is practical. That's what everybody is aiming for, including another massive government-funded project called ITER in France. And fusion reactors will have to reach Q = 10 much more frequently than NIF can.
In some ways, these are academic milestones, which fusion experiments have nudged toward for decades. But given fusion's reputation for not ever getting there, it's an important proof of what's possible. Think a little bit more carefully before you repeat that oft-quoted snarky remark that fusion is the energy source of the future and always will be.
What does the NIF experiment mean for green power?
Not a huge amount, for a few reasons. For one thing, most commercial fusion energy projects are using various forms of magnetic confinement, not NIF's laser-based approach, so the engineering challenges are different. For another, NIF is a gargantuan, $3.5 billion national lab project funded to research nuclear weapons, not a project designed to produce reliable energy for the grid at the most competitive cost.
"Don't expect future fusion plants to look anything like NIF," said Princeton researcher Wilson Ricks in a post on X, formerly Twitter. Huge inefficiencies in NIF's lasers and in the conversion of fusion heat to electrical power mean its design is inherently impractical. In comparison, "magnetic confinement fusion holds some real promise," Ricks tweeted.
Lowering fusion's cost is critical to its success since it'll have to compete against zero-carbon alternatives like today's fission-based nuclear reactors that can generate a steady supply of power and renewables like wind and solar that are cheaper but intermittent.
"Fusion's first competitor is fission," researchers at the Princeton Plasma Physics Laboratory concluded in an October research paper, not yet peer reviewed, that assesses fusion's prospects on the electrical grid. They expect that if fusion's high costs can come down enough, it could replace the need for future fission plants, and if lowered further, could also compete against the combination of solar and energy storage.
NIF is a big, complicated site. If fusion power plants can be built in cheaper, smaller units that are more like something coming off a factory line, production costs should decrease. That's thanks to a phenomenon called Wright's Law, the experience curve or the learning curve, which has steadily lowered costs for solar and wind. The bigger and more customized a fusion plant is, the less costs will drop and the less competitive fusion will be.
Are there some less direct benefits from NIF's results?
Yes. Scientists could benefit somewhat from the NIF experiment by updating fusion physics models to account for the fact that it's supplying its own heat instead of relying on external sources, said Andrew Holland, chief executive of the Fusion Industry Association, an advocacy group for the industry.
And the attention could help, too, especially given longrunning skepticism about fusion energy.
TAE Technologies CEO Michl Binderbauer called NIF's result "a huge stepping stone into the dawn of the fusion age," and said it's an important illustration that fusion energy really is plausible.
Investors have noticed, too. Downloads of the Fusion Industry Association's annual report, which details the $4.8 billion in venture capital investments in fusion energy startups, increased tenfold after the first NIF achievement was announced, Holland said. Many of those requesting it are from investment firms, he added.
How does fusion work at NIF?
NIF triggers fusion using 192 powerful infrared lasers with a combined energy level of 4 megajoules — about the same as a two-ton truck traveling at 100 mph. That's converted first into 2 megajoules of ultraviolet light, then into X-rays that strike a peppercorn-sized pellet of fusion fuel.
The intense X-rays cause the outer layer of the pellet to blow off explosively, compressing the pellet interior and triggering fusion. The heat from that fusion sustains the reaction until it runs out of fuel or becomes lopsided and falters.
The National Ignition Facility at Lawrence Livermore National Laboratory is the size of three football fields.
Nuclei? Hydrogen? Catch me up on atomic physics, please
Sure! Here's a quick refresher.
Everything on Earth is made of tiny atoms, each consisting of a central nucleus and a cloud of negatively charged electrons. The nucleus is made of neutrons and positively charged protons. The more protons in the nucleus, the heavier the element is.
Hydrogen usually has one proton and one electron. An unusual variety called deuterium has a neutron, too, and using nuclear reactors or fusion reactors, you can make a third variety called tritium with two neutrons.
Chemical reactions, like iron rusting or wood burning, occur when those positive and electrical charges cause atoms to interact. In comparison, nuclear reactions occur when the nuclei of atoms split apart or join together. Here on Earth, it's harder to marshal the required forces to get nuclear reactions to take place, which is why it's easier to make a steam engine than a nuclear bomb.
When you heat atoms up enough, they get so energetic that the electrons are stripped loose. The resulting cloud of negatively charged electrons and positively charged nuclei is called a plasma, a more exotic state of matter than the solids, liquids and gases that we're used to at room temperature here on Earth.
The sun is made of plasma, and fusion reactors need it, too, to get those hydrogen nuclei to bounce around energetically enough. A convenient property of plasmas is that their electrically charged particles can be manipulated with magnetic fields. That's crucial to many fusion reactor designs.
What do you use for fusion fuel?
NIF and most other fusion projects use the two heavy versions of hydrogen, deuterium and tritium, called DT fuel. But there are other options, including hydrogen-boron and deuterium-helium-3, a form of helium with only one neutron instead of the more common two.
To get deuterium and tritium to fuse, you need to heat a plasma up to a whopping temperature of about 100 million degrees Celsius (180 million degrees Fahrenheit). Other reactions are even higher, for example about a billion degrees for hydrogen-boron fusion.
Deuterium can be filtered out of ordinary water, but tritium, which decays away radioactively over a few years, is harder to come by. It can be manufactured in nuclear reactors and, in principle, in future fusion reactors, too. Managing tritium is complex, though, because it's used to boost nuclear weapon explosions and thus is carefully controlled.
How do you turn that fusion reaction into power?
The deuterium-tritium fusion reaction produces fast-moving solo neutrons. Their kinetic energy can be captured in a "blanket" of liquid that surrounds the fusion reactor chamber and heats up as the neutrons collide.
That heat is then transferred to water that boils and powers conventional steam turbines. That technology is well understood, but nobody has yet connected it to a fusion reactor. Indeed the first generation of fusion power reactors being built today are designed to exceed Q=1, but not to capture power. That'll wait for the pilot plants that are expected to arrive in the next wave of development.
Is fusion work funded by the government or the private sector?
Both. NIF is funded by the US government's nuclear weapons program. Government funding also pays for the Joint European Torus in the UK and ITER in France, both of which are more closely aligned with the goal of fusion energy generation.
But increasingly fusion energy is privately funded. Investors have poured $4.8 billion total into fusion energy startups, of which $2.8 billion arrived in the last year, according to the Fusion Industry Association's annual report published earlier in 2022. Most of that went to Commonwealth Fusion Systems, a startup that spun out of MIT and raised more than $1.8 billion in a funding round in 2021.
The government is now helping the private sector, too. The US Energy Department in September 2022 announced a Milestone Program that provides up to $50 million to build fusion energy pilot plants. The Biden administration, a fusion proponent, said in November 2022 that fusion energy is one of five key approaches to halve carbon emissions by 2030 and reach net zero emissions by 2050.
"Uncle Sam is getting serious," said Holland of the Fusion Industry Association. NIF's achievement is "a pass-the-torch moment, where it goes from science and national labs to the commercial sector."
How is fusion different from fission?
Fission, which powers today's nuclear reactors, is the opposite of fusion. In fission, heavy elements like uranium split apart into lighter elements, releasing energy in the process.
Humans have been able to achieve fusion for decades with thermonuclear weapons. These designs slam material like uranium or plutonium together to trigger a fission explosion, and that provides the tremendous energy needed to initiate the secondary and more powerful fusion reaction.
In bombs, the process occurs in a fraction of a second, but for energy production, fusion must be controlled and sustained.
Do fusion reactors create radioactive waste?
Yes, generally, but it's not nearly as troublesome as with fission reactors. For one thing, most of the radioactive emissions are short-lived alpha particles — helium nuclei with a pair of protons and a pair of neutrons — that are easily blocked. The fast-moving neutrons can collide with other materials and create other radioactive materials.
Fusion reactors' neutron output generally will degrade components, requiring periodic replacement that could require downtime lasting perhaps a few months every few years. It's vastly easier to handle than the high-level nuclear waste of fission power plants, though.
Hydrogen-boron fusion is harder to achieve than deuterium-tritium fusion, but part of its appeal is that it doesn't produce any neutrons and attendant radioactive materials. The most prominent company pursuing this approach is TAE Technologies.
What are the safety risks of fusion power?
Fusion power plants don't have the meltdown risks that have caused problems with fission reactors like the Fukushima and Chernobyl sites. When a fusion reaction goes awry, it just fizzles out.
But there still are significant operational issues that you'll see at major industrial sites, including a lot of electrical power and high-pressure steam. In other words, the big problems are more like those you'd find at an industrial site than at one of today's fission nuclear power plants.
So there are real advantages to fusion. NIF's work helps show that there's a future for fusion energy. But there's still a very long way to go.